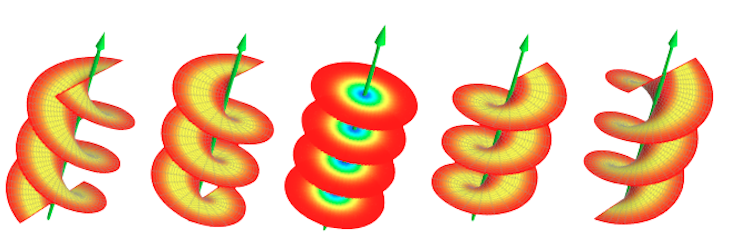
It's a question I'm sure was keeping you up at night: Can you make an object spin with a sound wave? The answer, generally speaking, used to be no. Now, though, mechanical engineers have taken a look at what their colleagues who play with lasers can do, and having seen the light, they copied it. And with that, spinning objects with sound waves has been achieved… but only in simulations.
Is it really that hard to make things spin?
To get an idea of why making things spin with sound waves is difficult, picture a tube that holds a turbine. Normally, to make the turbine spin, a fluid would flow past the blades of the turbine. The force of the fluid on the blades imparts a torque, which sets the turbine spinning. If we replace that flow with a pressure wave (like a sound wave), the fluid moves back and forth. So the local motion will first impart a torque that is clockwise and then one that is counterclockwise. The result is a rocking motion.
More fundamentally, the wave carries linear momentum but not angular momentum (specifically, it's orbital angular momentum, but we'll drop the "orbital"). Something that spins has angular momentum. In the turbine example, the total angular momentum cannot change. If the wave has no angular momentum and the turbine has no angular momentum, nothing will change.
(Flowing fluid doesn't have angular momentum, either, but it can set the turbine spinning. This works because the fluid will form vortices after it has passed the turbine. The vortices carry angular momentum with the opposite spin to the turbine so that the total angular momentum remains zero.)
This is all well-trodden ground. But until recently, no one was sure that sound waves could carry this type of angular momentum. Even assuming they could, we had no idea how to generate a sound wave with angular momentum. So the first step the researchers took was to show that sound waves could carry angular momentum. Having done that (the bulk of the thinking work), it was time to figure out how to generate the waves.
First, make a phonon dizzy…
The trick is to make mechanical waves spin. To visualize this idea, you need to understand the idea of a wavefront. For example, let's take a sound wave traveling in the air. A sound wave consists of high pressure and low-pressure regions that move through space. If we could freeze time, we could look down on the frozen sound wave and draw a line where the pressure is highest. This line (which is usually a curve) is at right angles to the direction that the wave is traveling. If we unfreeze time for an instant and then refreeze it, we'll find that the line has advanced at the speed of sound to a new position.
Our picture of wavefronts can be expanded to three dimensions. Here, the line of high pressure becomes a plane of high pressure that advances through space at the speed of sound.
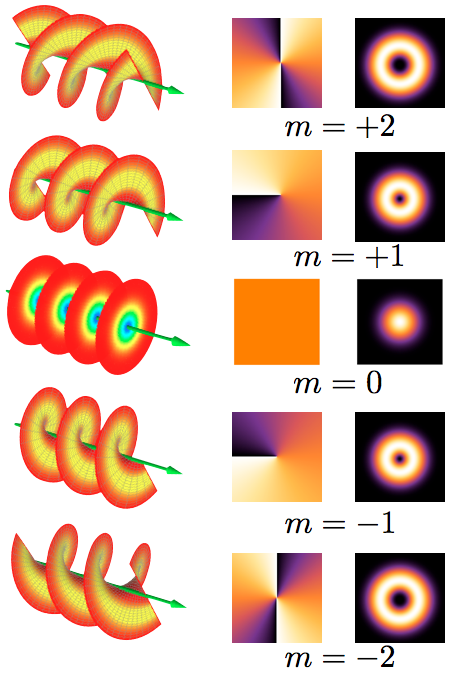
This wavefront describes the wave’s momentum and angular momentum. For a wave with angular momentum, the wavefront is no longer a line (or a plane). Instead, the surface is a helix (like an Archimedes screw). The wave still travels at right angles to the wavefront surface, but it is now a spiral that circles a central axis. If you were to take a single bit of wavefront and track it, you would find that it corkscrews along that axis.
What happens at the center of the corkscrew? At that location, the wavefront has no single value. The center has to be a location of simultaneously high and low pressure. The Universe doesn’t like self-contradiction, so it takes the average, and the sound wave at the center has no amplitude.
Then make your phonon ride a rollercoaster
A wave with a helical wavefront can set objects spinning, leaving us with a big question: How do we generate such a wave? To solve this problem, the researchers looked at how helical waves are generated in light beams and then translated the idea to mechanical waves. In optics, a piece of glass with a spiral etched into it (called a spiral phase plate) does the trick. The glass is transparent, allowing the light to be transmitted, but it also introduces an angle-dependent delay because the speed of light is slower in glass than in air. The light enters with wavefronts that are simple planes and exits in a spiral.
The equivalent in mechanical waves is a pipe, with sound waves traveling in the wall of the pipe. The thin wall acts as a waveguide for the sound wave and slows its speed compared to an unconfined sound wave. To impart angular momentum, the wall diameter is suddenly increased, but in a spiral pattern. The spiral increases the wave’s speed in an angularly dependent way, leading to a corkscrew wave.
A wave in the wall of a pipe is probably not of much use. However, the researchers also show that if the pipe ends in a fluid, the sound waves exit the pipe and retain their angular momentum. That means the angular momentum can be put to work on objects.
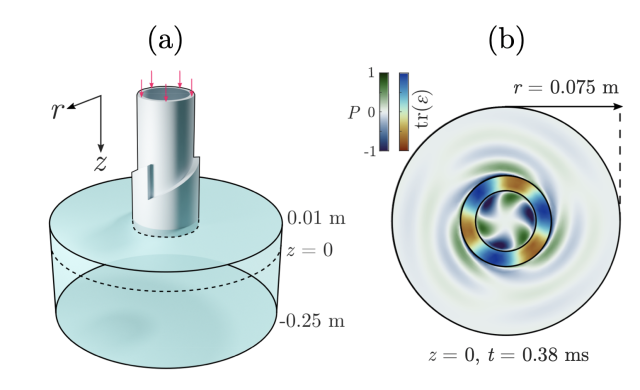
Where’s my spinning turbine?
At this stage, the researchers have only managed to perform simulations of the waves. I imagine that a demonstration is not that far away, but it's probably not easy to image a sound wave like this, so video evidence may be a way off.
In any case, it's important to understand that this system will not replace flowing fluids through turbines to generate power. Instead, it's more likely to find application in object manipulation. In optics, beams like these could be used to trap and impart rotation to free-floating particles. Angular momentum beams are also used for imaging. Sound waves with angular momentum will probably be used in a similar way.
Physical Review Letters, 2022, DOI: 10.1103/PhysRevLett.128.064301 (About DOIs)
"sound" - Google News
March 03, 2022 at 02:00AM
https://ift.tt/p7PCJnD
Twisting sound waves for new acoustic trapping and ultrasound techniques - Ars Technica
"sound" - Google News
https://ift.tt/DMxYkaf
Shoes Man Tutorial
Pos News Update
Meme Update
Korean Entertainment News
Japan News Update
No comments:
Post a Comment