If you’ve ever been in the presence of dark rain clouds and stormy weather, you’ve likely experienced the two most notable and shocking phenomena that accompany them: thunder and lightning. Whether from up close or afar, the lightning always looks the same, but thunder sounds tremendously different depending on your distance. Thunder — the sound resulting from the aftermath of lightning — typically sounds like a loud clap if you’re close by, with accompanying rumbles that last for a relatively short time period. From far away, however, it’s almost exclusively rumbles, and those rumbles are softer and more drawn out: lasting for a much longer period of time.
Does this bother you? Perhaps it should. It bothered Patreon supporter Rob Hansen, who wrote in and asked:
“Even as a child I knew thunder sounded different depending on how far away the lightning strike was. Last week I realized I didn’t know why. Close to the origin it’s a quarter-second of intense pressure, but as distances increase the sound stretches out into a multi-second low basso rumble… If the speed of sound in a given medium is constant, shouldn’t all the sound energy reach me at the same time? What’s the cause of this stretching-out phenomena?”
There are a couple of explanations you might consider, but only one is the actual culprit for this phenomenon. Let’s think about how it works.
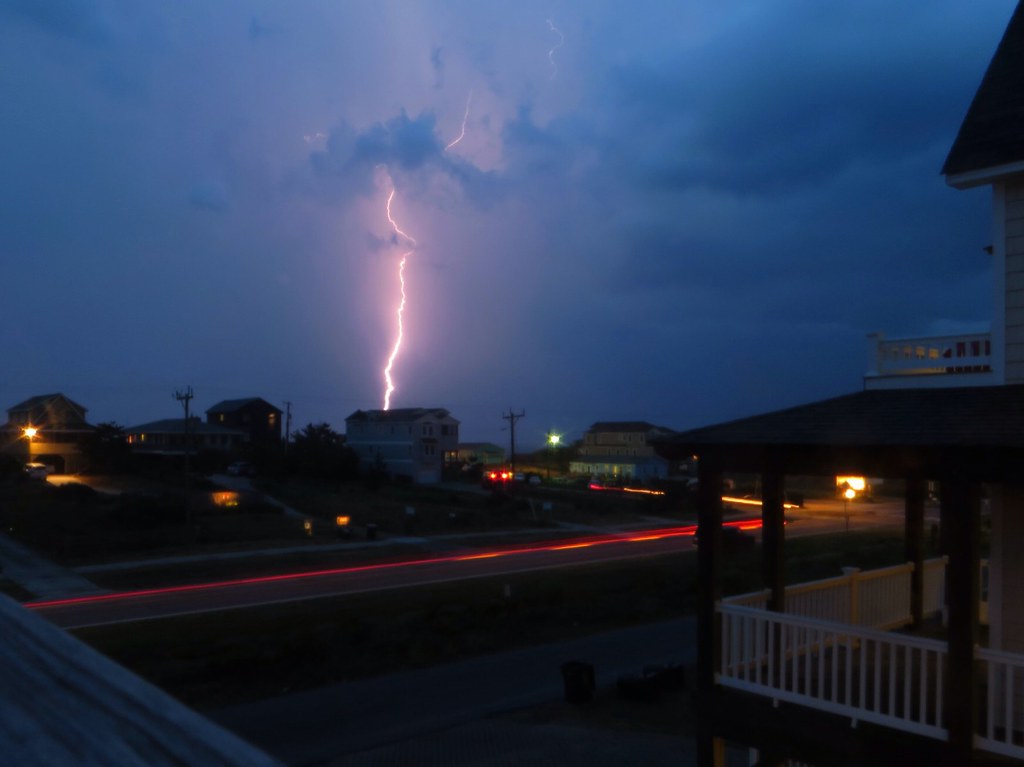
One well-known trick to approximate how far away a lightning/thunder event is involves counting the number of seconds it takes to hear the thunder’s first arrival after seeing the flash of lightning. Every three seconds of delay corresponds to approximately 1 kilometer (~0.6 miles) of distance.
Sound, quite simply, is a wave that travels through a medium: whether a gas (like air), a liquid (like water), or a solid (like the Earth). If you ever learned about seismic waves that travel through the Earth, you’ve learned about at least two types of wave:
- a longitudinal wave (or P-wave), which is a series of compressions and rarefactions, as though you took a stretched slinky and quickly “pulsed” it in the direction in which it was stretched,
- or a transverse wave (or S-wave), which makes a series of peaks-and-troughs, as though you took that same stretched slinky and quickly moved it back-and-forth perpendicular to the direction in which it’s stretched.
These two classes of waves have different speeds through any medium, and so you might imagine that the farther away you are, the bigger the difference in the arrival times of the different waves will be, causing the sound to “stretch out.”
This might be your first scientific thought, but unfortunately, you have to reject it. While the longitudinal P-waves can travel through solids, liquids, and gases, the transverse S-waves can only travel through solids. The “thunder” sound that you hear travels only through air, so with the absence of transverse S-waves, this explanation won’t help you.
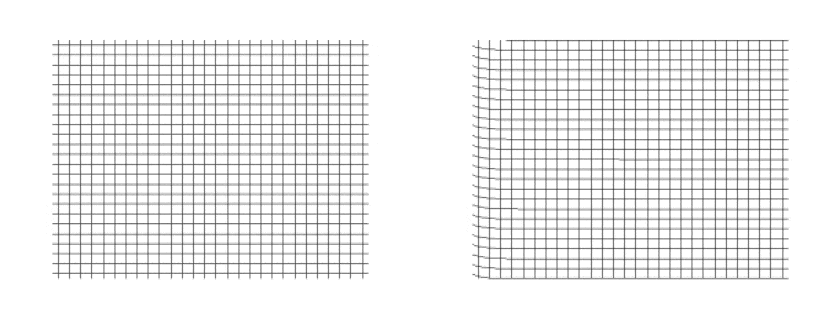
This side-by-side illustration shows a plane compression wave, or a longitudinal P-wave at left, alongside a transverse S-wave at right. While P-waves can travel through solids, liquids, and gases, S-waves can only travel through solids.
Instead, thunder is the sound produced as the result of a lightning event: the rapid exchange of tremendous amounts of electric charge over only a tiny fraction-of-a-second. Up to several coulombs of charge (that is, more than ~1019 individual electrons) are typically exchanged in a lightning strike, either from cloud-to-cloud or cloud-to-ground here on Earth. As the electrons rapidly flow, they heat up and even ionize the air molecules around them, creating a very brief “plasma” state and causing the air to rapidly expand.
That rapid expansion pushes the surrounding air rapidly outward, and then — because it’s created a low-density region along the collimated path where the lightning strike occurred — the air rushes back in to fill the temporary vacuum-like state that was created. This rapid expansion-and-contraction creates a shockwave: a pressure wave, where the alternating phenomena of air compression (i.e., the “denser” parts) and air rarefaction (i.e., the less dense, or more “expanded” parts) occur, propagating through the air.
This movement of air pushes particles in a chain reaction, and those compressions and rarefactions will travel through the air until they reach your eardrum, where the pressure changes cause your eardrum to vibrate, leading to the phenomenon you experience as the sound of thunder.
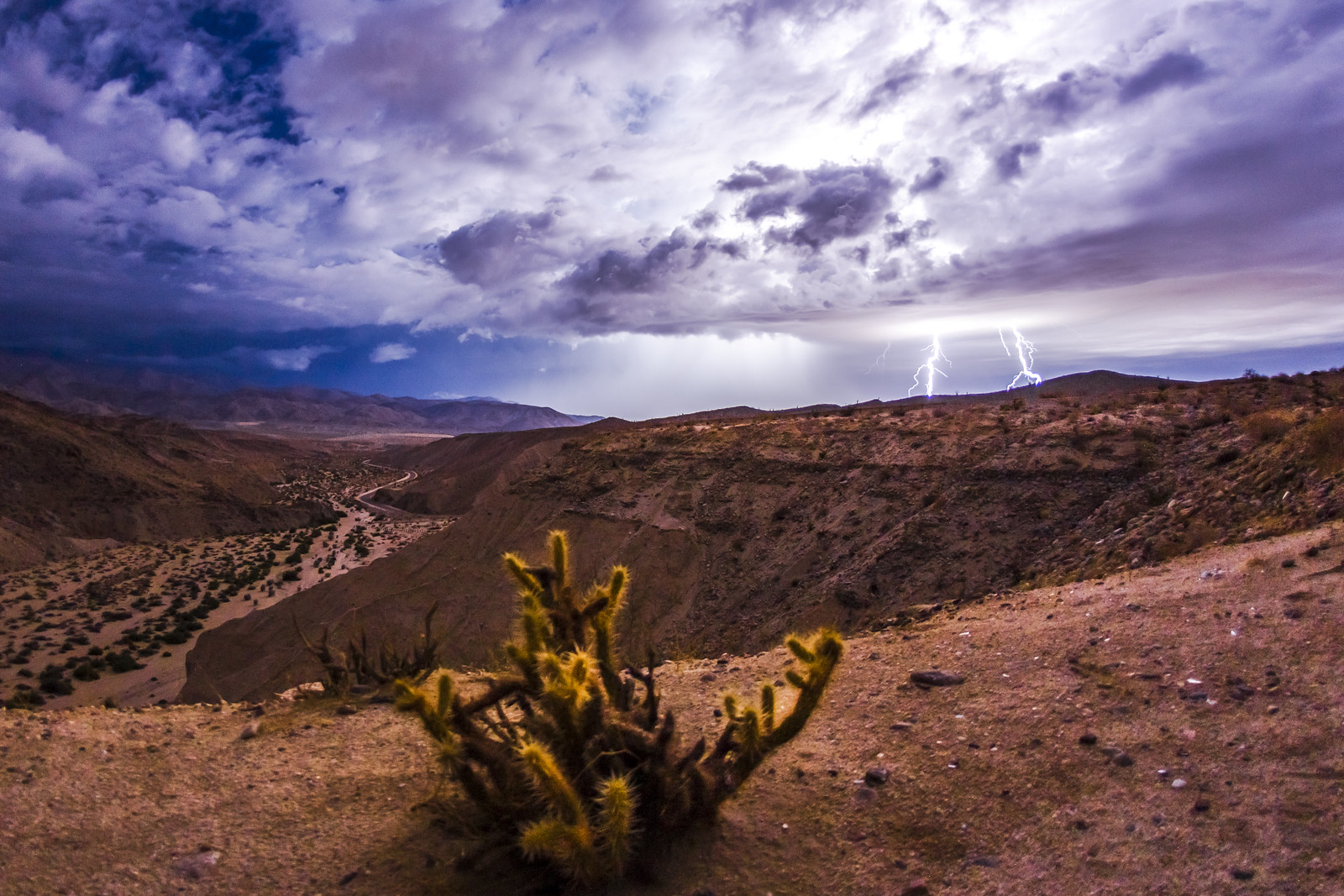
Although a lightning strike is simply the exchange of charged particles, it superheats the air to some ~30,000 degrees Celsius, resulting in the creation of a pressure wave that propagates through air at the speed of sound. When that pressure wave collides with your eardrum, that’s what determines the sound you hear. This includes all the “lossy” and stretching effects that occur to those pressure waves along their journey from the source to the receiver.
You might wonder, then, if there isn’t some variation in the speed of sound that might affect how different observers hear it.
For example, what if the speed of sound were frequency-dependent? In other words, what if “bass notes” (i.e., low-frequency sound waves) traveled at different speeds than intermediate or high-pitched (with higher frequencies) notes?
This is an effect that’s of tremendous practical importance in low-density conditions, such as in the atmosphere of Mars. In the Martian atmosphere, high-frequency sound travels about 4% faster than low-frequency sound, implying that the arrival time of the various sounds would get stretched out the farther away you are from the source of the sound.
But on Earth, the speed of sound barely changes with frequency, as our atmosphere is much thicker than the Martian atmosphere. From an ultra-low frequency of 10 Hz (below the threshold of human hearing, which begins at 20 Hz) to a modest frequency of 100 Hz, the speed of sound changes only by 0.1%, and then from 100 Hz all the way up to the highest frequencies that humans with undamaged hearing can hear (about 20,000 Hz), the speed of sound remains constant. This frequency-dependence is negligible on Earth, and cannot be responsible for the “stretching” effect of the sound.
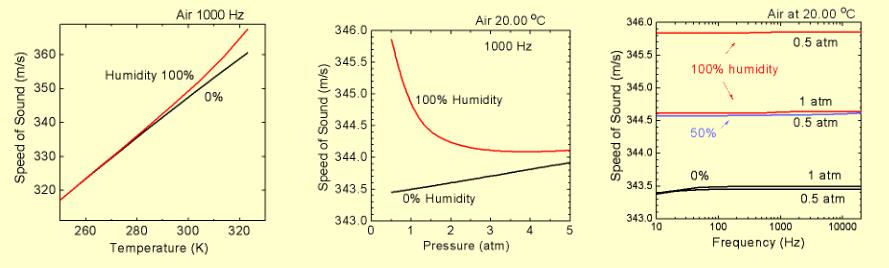
The three graphs here show how the speed of sound varies with temperature (left), atmospheric pressure (center), and frequency (right). Although differing humidities lead to different dependencies, the scale of the y-axis of the three graphs shows that speed of sound is very sensitive to changes in temperature, but not to changes in pressure or frequency.
If you want to hear the differences for yourself, there’s a website from the Canadian Government that’s provided a recording of the same thunderous sound arising from a cloud-to-ground lightning strike, as you’d hear it at a variety of distances:
If you did a frequency analysis of the waves that arrive, you might find — perhaps to your surprise — that the farther away you are, the less you hear the higher-pitched sound waves.
This is a real effect, but it’s not because waves are traveling faster or slower through the Earth’s atmosphere depending on their frequencies. Instead, it’s because of the motion of the air particles: it’s easier to compress-and-rarify particles only a few times per second than it is to compress-and-rarify them many times per second. In other words, higher frequency sound waves get absorbed and dissipated by the medium (even in Earth’s atmosphere) they’re traveling through than lower frequency sound waves.
Additionally, the presence of water in the atmosphere — humidity — also absorbs sound waves, and in a frequency-dependent way. This explains why you hear more of a bass-sounding rumble, exclusively, from far away than from up close, but it still doesn’t explain why the more distant sound waves are stretched out to last about three times as long as the nearby sound waves.
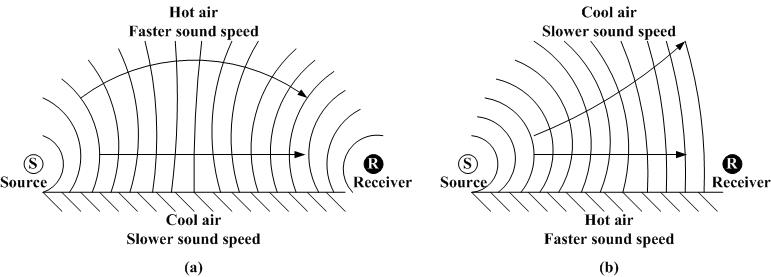
Owing to factors like temperature and density gradients, as well as varying wind speeds, sound waves will take varying amounts of time along different pathways to go from the source to the receiver. The farther away the receiver is, the more drawn-out in time the received sound will be.
However, there are three very real effects that do change the speed of sound through a medium like air: wind, density, and temperature.
Wind is an additive effect: if the wind blows away from you and toward the lightning source, the sound of thunder takes longer to arrive. If the wind blows toward you and away from the lightning source, the sound of thunder arrives more quickly. But wind doesn’t travel at the same universal speed at all locations, and this is important because a lightning strike doesn’t affect simply a point, but rather a “line” in three-dimensional space. If the wind speed differs along your line-of-sight to different points along the lightning bolt’s “line,” the sound arrives sooner-or-later depending on the wind’s relative speed between you and the lightning strike itself.
Density usually varies with altitude: the higher you rise in altitude, the less dense the air, while the closer you are to sea level, the denser the air. This means that the component(s) of the lighting bolt that occurs at higher altitudes typically travels slower (and hence, arrives later) than the component(s) of the lightning bold that occurs at lower altitudes (which arrive earlier). For a cloud-to-ground strike, particularly if the clouds are at relatively high elevations above the ground, this effect can contribute to the “delay” effect.
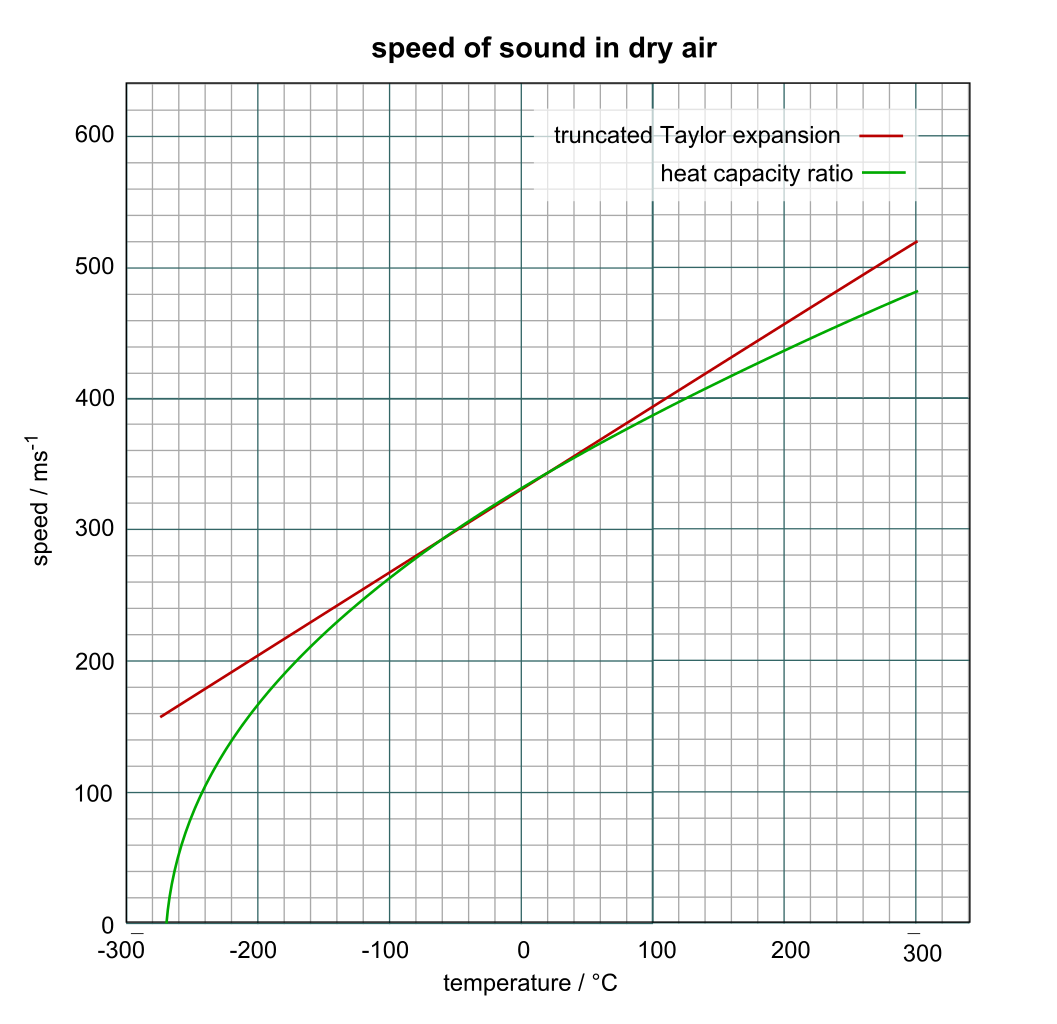
The green line and red line on the graph show the relationship between the speed of sound in 0% humidity air on Earth as a function of air temperature. The red curve is an easy-to-calculate approximation; the green curve is more exact, but both match up very well over realistic air temperatures found on Earth.
But even wind and density, combined, can’t account for the majority of the observed delay. The largest effect on the speed of sound is temperature, where higher temperature air corresponds to a greater speed of sound through that medium. Even just a temperature change of 1 °C (1.8 °F) on Earth changes the speed of sound by 2.2 kph (1.3 mph). If you’ve ever been in a thunderstorm, you’ve no doubt felt the winds of varying warmer-and-colder temperatures that blow under those conditions, as thunderstorms usually arise when warm-and-cold air interact.
Even small temperature variations of just a few degrees, when accumulated over distances of a few kilometers or miles, can stretch out the arrival time of a burst of sound waves by several seconds. When combined with winds and density variations, these three effects — together — can explain the entirety of why the arrival time of the sound appears to get stretched out more severely the farther away you are from the lightning strike itself.
Travel the Universe with astrophysicist Ethan Siegel. Subscribers will get the newsletter every Saturday. All aboard!
However, there’s one aspect of thunder that isn’t explained by this: why sometimes you hear a loud, sharp “thunderclap,” and other times there’s no such feature, but only a low, sustained rumbling sound.
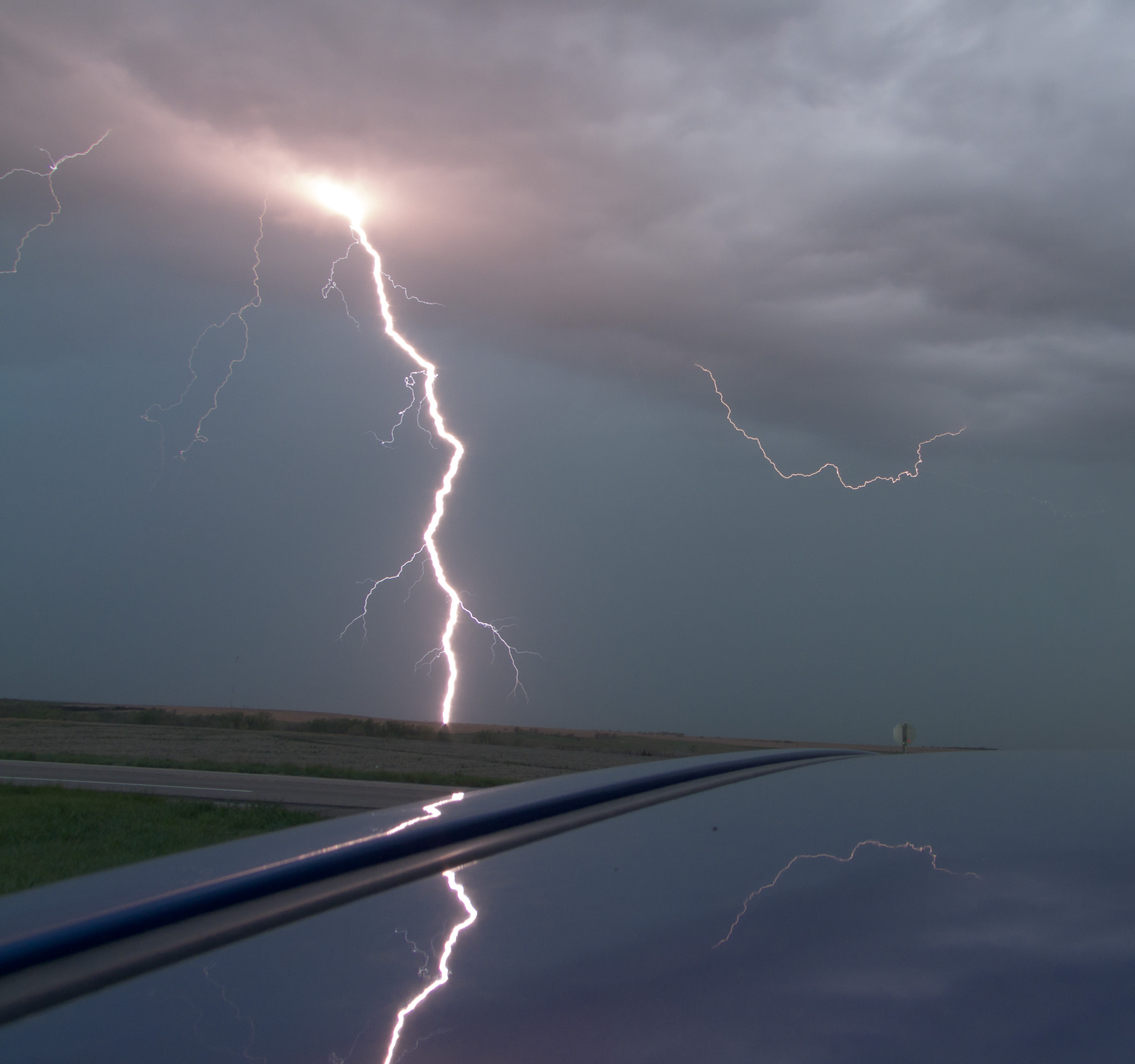
The bright, prominent lightning bolt in the foreground is an example of vertical, cloud-to-ground lightning. Bolts like this are usually no more than 5 kilometers (3 miles) in length, and are often accompanied, especially nearby, by a loud and swift thunderclap sound.
One of the things you have to remember is that even though what we see as a “lightning bolt” is generally a one-dimensional line (sometimes with branches), this line exists in our three-dimensional space. Exchanges can be modeled as perfectly vertical (cloud-to-ground) or perfectly horizontal (cloud-to-cloud), but there will often be a depth to that line as well: where one portion of the lightning bolt is closer to you, the observer, and one portion is farther away from you.
When cloud-to-ground lightning occurs, that “depth” portion is fairly negligible. Cloud-to-ground lightning is usually fairly vertical: traveling in an imaginary line connecting the cloud to the center of the Earth. Why? Because that’s literally the path of least resistance for the electrical current to travel: the shortest path down to the Earth’s surface.
As a result, the sound waves resulting from a cloud-to-ground lightning event:
- don’t have to travel through the extremely humid clouds, so their high-frequency sounds aren’t dampened by very much,
- arrive over a very short time interval, as the distance from the lightning bolt to the observer is very close to equal across all points of the lightning bolt.
This is why, when you have vertical cloud-to-ground lightning strikes, the sound they produce is a sharp “thunderclap” sound, followed by only a brief rumble: determined by the time difference between when the sound from the bottom of the bolt reaches you versus the sound from the top.
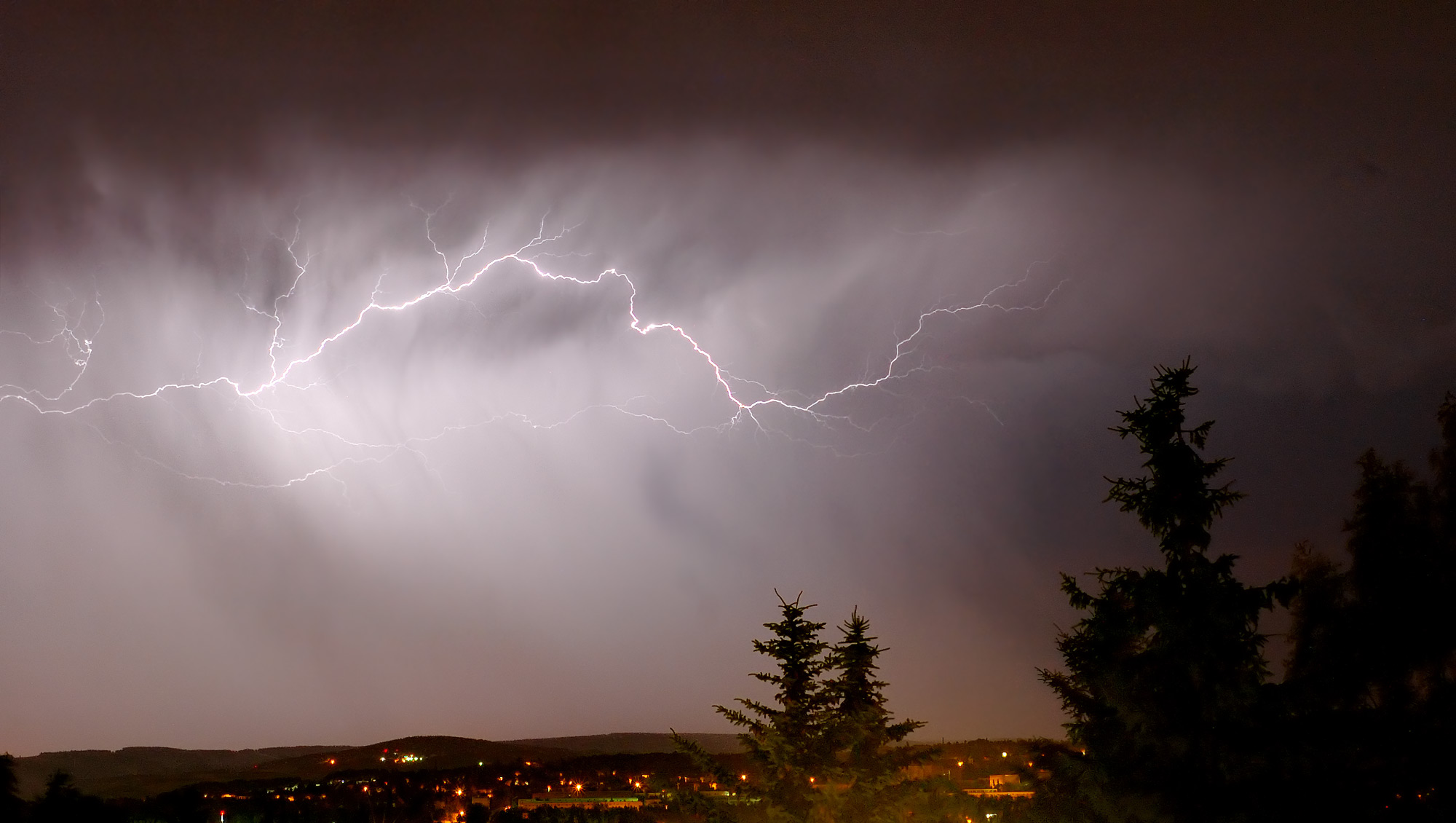
This horizontal lightning bolt is an example of cloud-to-cloud lightning, as captured over Zwickau, Germany. Whereas vertical lightning bolts are typically no more than 5 kilometers (3 miles) long, horizontal ones can be much longer on occasion.
On the other hand, cloud-to-cloud lightning is generally a one-dimensional, horizontal line that isn’t all at the same distance; its “depth” orientation is random. One end of the lightning bolt will generally be closer to the observer, while another end will be more distant. While vertical cloud-to-ground lightning bolts are usually no more than 3-5 kilometers long (2-3 miles, roughly), horizontal cloud-to-cloud lightning bolts can be much, much longer. In fact, an analysis of a 2020 storm uncovered a record-setting horizontal lightning bolt of an incredible — and this is not a typo — 477 miles ( kilometers) in length!
For cloud-to-cloud lightning bolts, the sound is mostly a rumble, as the higher-frequency “crack” of a thunderclap gets largely drowned out by the humidity within the clouds themselves. The duration of the sound will still be lengthened, dependent on your distance from the lightning, by the effects of wind, by differences in air density, and by the varying speed of sound based on temperature. However, there’s also the difference in distance between the “near end” and the “far end” of the cloud-to-cloud lightning as those sounds travel to the observer.
The duration of the thunder that you hear is not only determined by how much the sound is “drawn out” by propagation effects, but also by the length and geometrical orientation of the bolt itself relative to where you’re located.
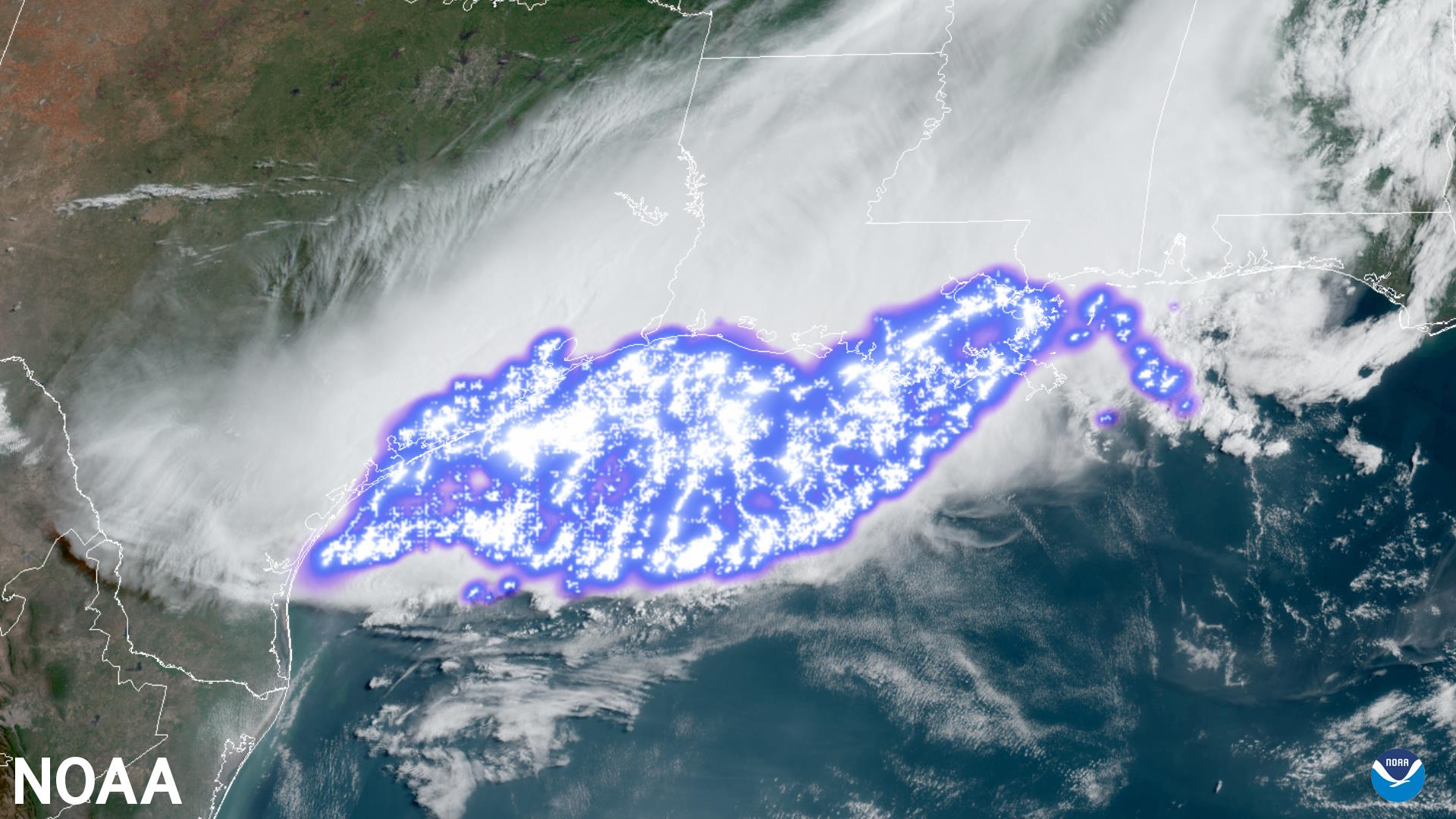
Lightning as seen from the Geostationary Lightning Mapper on NOAA’s GOES-16 satellite from April 29, 2020. One of the lightning flashes within this thunderstorm complex was found by the World Meteorological Organization to be the longest flash on record that covered a horizontal distance of 477 miles.
Of course, there’s an upper limit to the duration of any thunderous sound that you hear, as the more distant a lightning bolt is from you, the lower the intensity of the sound waves that strike your eardrum. Depending on the air conditions, thunder might be audible as far away as ~20 kilometers (~12 miles) if conditions are favorable, or only as distant as ~8 kilometers (~5 miles) if they’re not. Remember, the energy in a sound wave spreads out as the square of the distance from the source, so when you’re twice as far away, you only get one-quarter the sound intensity; when you’re 10 times as far away, you only receive one-hundredth of the original sound intensity.
When you fold in the fact that the sound gets drawn out over a longer period of time and dampened the farther away you are as well — due to wind, density, humidity, and temperature effects — this combines to make more distant lightning strikes:
- quieter,
- more “rumbly” and less “thunderclappy,”
- and audible over longer timescales.
If you want to attribute the lengthening sound of thunder with distance to one primary effect, it’s air temperature. But the more comprehensive truth is that all of these effects play a role, causing sound waves to bend, get absorbed, and impinge on your eardrum with varying intensities and frequencies over time. Just remember the most important part: the closer the thunder, the more urgently you should get indoors. After all, about 1-in-15,000 people will be struck by lightning at some point during their lifetime. Don’t let it be you!
Send in your Ask Ethan questions to startswithabang at gmail dot com!
"sound" - Google News
April 07, 2023 at 01:00PM
https://ift.tt/WrZhgd5
Ask Ethan: What explains the delicate sound of thunder? - Big Think
"sound" - Google News
https://ift.tt/NnTXZJw
Shoes Man Tutorial
Pos News Update
Meme Update
Korean Entertainment News
Japan News Update
No comments:
Post a Comment